Nocturnal Mammals
From Comparative Physiology of Vision
Nocturnal Mammals are primarily active at night and rest during the day. [1] Functioning at night in most nocturnal mammals requires the ability to see in dark conditions. This is achieved through anatomical differences such as a Tapetum lucidum which many nocturnal animals possess, and differences in various structures of the eye, such as large pupil sizes.[1] [2]
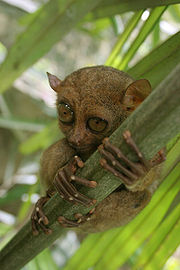
Contents |
General Anatomy
Pupils
Nocturnal mammals tend to have larger eyes as well as larger pupils that can open widely in low light. Having a larger eye and larger pupil allows a nocturnal mammal to receive more incoming light thus gather more photons due to the larger surface area exposed. Thus, the large pupil helps to receive as much light as possible in low light conditions. [3] Additionally, at lower light levels it is harder to distinguish edges and borders and thus shapes because there is a decrease in contrast in low light conditions. The way that nocturnal mammals adjust for this is by increase “quanta” or light capture by having large pupils and short focal lengths. [4]
Due to this increased sensitivity the animal needs to protect its eyes from the high intensity light that it could possibly experience in the day. The way that nocturnal or diurnal mammals accommodate such a wide range of light intensities is through having a slit pupil. Slit pupils let in less light. Light can further be reduced by having a vertical slit and partially closing the eyelids. [1]
Due to these very large pupils nocturnal mammals have a small depth of field. This is due to the pupil being so large in comparison to the focal length. This can result in chromatic defocus. This chromatic defocus is due to light hitting a medium and differing wavelengths travelling at different speeds causing chromatic defocus or chromatic abberation.
This chromatic defocus can cause blurry images. However, many animals have adapted by having multifocal lenses with concentric zones of differential focal lengths. These concentric zones focus a specific spectral range onto the retina allowing for better resolution of the image. Due to multifocal lenses and the nocturnal mammals eye being highly sensitive to bright light circular pupils would not work. If the animal had circular pupils then if the animal experienced intense light during the day when the pupil constricts it would cut out many of the zones of differential focus creating a very blurry image. Thus, slit pupils allow for use of the entire diameter of the eye and each zone of differential focus even when high intensity light is encountered. This allows nocturnal mammals to function during the day if necessary. [5]
Additionally, slit pupils are much more efficient at opening and closing. In species with round pupils the pupil opening and closing is controlled by circular cilary muscles. However, this limits the diameter in which the pupil can dilate to and is not an efficient system for opening and closing the pupil aperture quickly. In animals with slit pupils the pupil opening and closing is controlled by two cilary muscles. The efficiency is due to the way that the cilary muscles bunch as they contract. These muscles are much more efficient at closing in either direction as opposed to radially. [6]
Rods and Cones
Rods are sensitive to low light conditions. The retina of nocturnal mammals have many more rods than cones. There are very few cones compared to the amount of rods present in the nocturnal mammals retina. The rhodopsin in rods is very sensitive to light, which enables it to sense low levels of light. In animals that are functional during the day many have both rods and cones. In these animals the rhodopsin in the rods breaks down or is “bleached” faster than it can be regenerated making the rods useless and vision is primarily sensed by the less sensitive cones. However, in nocturnal animals rods predominate and in low light conditions rhodopsin is regenerated fast enough for the animal to effectively use these rods. Cones need higher intensity photons to be excited and thus are rendered essentially useless in low light conditions. However, cones allow for greater visual acuity. Thus, in nocturnal animals visual acuity is sacrificed for being able to see well at night. [7]
Some animals that can function during the night are also capable of 24 hour activity. This ability is conferred by having enough cones for a secondary image forming system. Essentially, having enough cones to produce an image during the day. In these conditions rods become saturated with light bleaching rhodopsin and cones are relied on for image transduction. [8]
In addition to nocturnal mammals having more rods than cones in comparison to non-nocturnal mammals the rods themselves are different. Each rod acts as a lens by packing its heterochromatin in the center of the nucleus as opposed to on the periphery. This is unusual because most other organisms have heterochromatin lining the inner membrane of the nucleus. This arrangement transforms the nucleus into a small lens that both collects and funnels light through the retina. If the nucleus did not have this conformation it would instead scatter light. The reason this is so important is because the light collecting portions of the rods and cones are at the base of the retina and light must pass through a layer of neurons to reach the light sensitive portion of the photoreceptor cell. Thus, by inverting the heterochromatin the rod acts as a lens that focuses and directs light to the photosenstive portion of the rod cell. This formation allows for efficient collection of light allowing the animal to see at extremely low light levels. [9]
Tapetum Lucidium
The Tapetum is a thin reflective membrane at the back of the eye (choroid) that reflects the light that has already passed through the retina. This allows the light that was not absorbed by the photoreceptor cells on the initial transmission of light to be reflected by this membrane and given another chance to be absorbed by the photoreceptor cells. If the light is not absorbed upon reflection it will be reflected back out of the eye through the pupil. This explains why many nocturnal mammals appear to “glow” when light is shone on them in the night. [10] The composition of the tapetum can vary widely between species. In some species it is a regular array of crystals in others it is composed of fibers. Many carnivores tapetum is made up of zinc-cystiene. The tapetum in lemurs is made up of riboflavin crystals.The composition of the tapetum can vary widely between species. In some species it is a regular array of crystals in others it is composed of fibers. Many carnivores tapetum is made up of zinc-cystiene. The tapetum in lemurs is made up of riboflavin crystals. [11]
Lenses
Nocturnal mammals tend to have thicker, larger and more highly curved lenses. The focal length is extremely short, in some mice the lens almost touches the retina. The combination of short focal length and wide aperture results in a low focal ratio and high light gathering ability. In this way resolution of the image is sacrificed for the ability to gather light. When the whole lens is used images are often blurry because of chromatic abberation. However, many mammals adjust for this by having multifocal lenses. Depth of focus in nocturnal mammals is small because the “f-value” is small. (f=focal length/ pupil diameter). As stated nocturnal mammals have large pupils and very short focal lengths. Because the depth of focus is so short even small amounts of defocus can blur an image. As stated previously many nocturnal mammals have multifocal lenses to reduce chromatic abberation. Additionally in these same animals lenses are often very thick, close to being spherical. [5]
Nocturnal mammals have such short focal lengths and large lenses because the optics of pupil diameter and focal length determine image brightness. By having larger eyes, larger pupils, and a larger lens more light is collected and the image projected on the retina is brighter. However, the trade-off is that the image projected may be brighter but the image size projected on the retina is smaller. [12]
Eye and Neck
Many nocturnal mammals have eyes that are so large that they are not able to move them and instead must rely on moving their head back and forth and swiveling their necks. Many nocturnal mammals have their eyes fixed in their skulls. The reason that the eye is in a fixed position in the skull is because the eye is so large in comparison to the socket that it must remain in a fixed position. Thus, to compensate for deficient eye movement many nocturnal mammalian species have compensated for this by being able to swivel their heads to the far left and right in order to scan for possible predators or prey. For example, the tarsier with the largest eye size relative to body weight of any other mammal can swivel its head 180 degrees in either direction. [13]
Many mammals also have a nictitating membrane or membrana nictitans. This is essentially a "third eyelid" that helps with the function of the eye. Specifically, this membrane helps to protect the cornea and redistribute and add to tear flow. [14] Additionally, this membrane can be advantageous in other ways as well. This membrane can help to mute the bright glare from the tapetum lucidium that can give their location away if not masked. Additionally, this membrane can help to protect the eye from high intensity light. [15]
Unique Visual Optics
Tapetum lucidum
The Tapetum Lucidum is a reflective layer of tissue in the back of the eye, directly after the set of neurons, rods and cones which sense the light. The purpose of the tapetum lucidum is to reflect light onto the rods and cones for better vision in low light situations. The tapetum lucidum is what is responsible for the eye shine we see in many animals, such as cats and dogs.[16] Not all nocturnal animals have a tapetum lucidum, for example, the Tarsier lacks a tapetum. Humans do not have a tapetum lucidum.
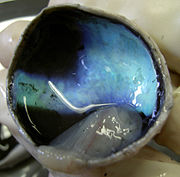
Anatomical Variants
There are four defined anatomical variants of the Tapetum Lucidum. [16]
- Retinal Tapetum – Lies within the retina
- Choroidal Guanine Tapetum – Tapetum contains crystal of guanine
- Choroidal Tapetum Cellulosum – Tapetum contains highly refractive crystals
- Choroidal Tapetum Fibrosum – Tapetum contains extracellular fibers
Ultraviolet Sensitivity
Ultraviolet (UV) sensitivity is not common among mammals. Research has shown that some mammals, such as the Degu, have the ability to sense UV. In this study, the UV sensitivity was shown to be caused by a shifted sensitivity of the blue cones, as opposed to the addition of a new cone specific to UV. [17]
Multifocal Lenses
Slit Pupils
Photo Transduction
content removed
Color Vision
Nocturnal mammals are generally color blind or dichromats. Mutations commonly found in nocturnal animals render the SWS gene nonfunctional and lead to a lack of S-cones. Mutations causing nonfunctional SWS genes have been found in many nocturnal primates such as the owl monkey and the bushbaby. In nocturnal animals with dichromatic vision, the short wave cone has often evolved sensitivity to UV light, in addition to the blue light spectrum this cone responds to in humans. Rats are an example of this dichromacy, they have a mutated S-cone that detects UV light and M-cones. [21] The ability to detect short wave lengths does not provide an evolutionary advantage at night, consequently nocturnal animals have evolved a more style of visual perception that is advantageous in their environment. For example, in rats 99% of the retina is rods and only 1% cones, it is believed these figures are proportional to the evolutionary importance of color vision in nocturnal species. Color vision in bats is an exception to the norm. They have S-cones which have been specialized, allowing them to detect UV light, which is most abundant at dusk and dawn when bats are most active. They also have M/L cones, with more of a long wave character, which is especially sensitive to red colors. This is thought to provide bats with an advantage in locating ripe fruits.[22]
Mammals that have rods and one type of come use their rods in dim light and their cones in brighter light conditions.[23] Most mammals are dichromats containing one gene for medium or long wavelength sensitivity (M/LWS) and one for short wavelength sensitivity (SWS). M/LWS senses green/red and constitute about 90% of cones. SWS senses blue/violet associated wavelengths and constitutes about 10% of cones.. Certain mammals are known to be monochromats and are thus colorblind. These species include the flying squirrel, blind mole rat, and some cetaceans (whales). These species are known to have a loss of function in the SWS gene likely due to them not needing dichromacy because of their environments and thus experience relaxed selection for this gene.
From an experiment sequencing the opsin genes of multiple species of bat it was found that the M/LWS gene sequence was highly conserved across all species with consensus sequences in intron splicing sites, which is indicative of an intact gene. However, the SWS sequence was more divergent and there was loss of function of these gene in some species. Loss of function in this gene was more common among bats that had “high-duty-cycle” echolocation. This form of echolocation enables a bat the continuously send out a signal. Bats that have “low-duty-cycle” mostly still had a functional copy of the SWS gene. Bats that have “low-duty-cycle” echolocation send out a signal and have to wait for a response before sending out a signal again. Loss of function of this gene is likely due to relaxed selection for this gene. On the whole bats are exclusively nocturnal and have even developed extrasensory functions such as echolocation to assist in their function in low light conditions. Additionally, the retina of a bat is composed almost entirely of rods. Thus, whether bats have dichromatic vision is still debated. A few species of bats were found to have purported functional SWS and M/LWS genes while most others only had a functional M/LWS gene. [24]
Additionally, in bats it was found that the M/LWS gene had more L character than M. L or longwavelengths are associated more with the color red. This may be helpful in possibly identifying ripe fruits, giving some evolutionary advantage. It was also discovered that the SWS gene may have shifted to pick up wavelengths in the UV spectrum. UV light is more abundant at dawn and dusk. [22]
Not only in bats, but in other species as well, mutations that render the SWS gene nonfunctional are also common. These species include the owl monkey as well as the bushbaby (Both primates). Lack of S-cones is also common among other species of mammal that are nocturnal as well indicating that S-cones are not nessecary for those species exhibiting strong nocturnality and thus relaxed selection for this gene can lead to mutations and create a non-functional S-cone gene. In comparing the common rat to the ground squirrel it was found that the highly nocturnal rat had eight times more rods than the highly diurnal ground squirrel. However, it was also found that both the rat and ground squirrel had two types of cones and both species have dichromatic color vision. However, this color vision was found to be much better in the ground squirrel. [25]
As mentioned previously, the owl monkey (Aotus trigirgatus) is an example of a monochromatic nocturnal mammal. The ratio of rods to cones in the retina is overall about 50:1, while the ratio shifts anywhere from 93:1 in the far periphery down to 14:1 in the central retina. Many species of platyrrhine monkeys have a distinctive cone photopigment polymorphism, which suggests that two separate L and M (long and middle wavelength) genes evolved from a common evolutionary origin through a crossing over mechanism in catarrhine primates sometime after the catarrhine/platyrrhine divergence. Surprisingly, the nocturnal Aotus lacks any evidence of such polymorphism.[26] Evidence suggests that the Aotus have only one class of adaptable cone photopigment that absorbs light between the middle to long wavelengths (between the spectral range of 540-630 nm). Though it can be very difficult to prove that a specific cell type does not exist, researchers have found strong evidence suggesting that Aotus do not have a population of functional short-wavelength sensitive (SWS) cones in the retina. Though DNA hybridization analysis shows the species to contain a pigment gene highly homologous to the human SWS pigment gene, it is still unknown as to why the homologous SWS pigment gene is not translated.[26] The Aotus is also unique among most nocturnal mammals in that (like the aye-aye) it does not have a tapetum lucidum for increased light reflection and absorption by photoreceptor cells in the eye. While monochromacy is commonly found among nocturnal mammals, the aye-aye is a dichromatic mammal, having both M/L- and S-opsin genes still intact. These findings imply that dichromacy may still be advantageous among nocturnal primates for possible reasons such as avoidance and detection of diurnal predators during the day, and the ability to perceive color under moonlit conditions while foraging for food.[26] Though common among Lorisformes, L-cone monochromacy is very rare among terrestrial mammals, implying that the loss of the S-opsin occurred very early in the evolutionary history of this group.[27]
Motion Detection
There are essentially two modes of vision, phototropic and scotopic. Phototropic functions at high levels of illumination, relies on cones, and enables one to see colors and fine details. Phototropic vision is usually not functional at night due to the high levels of illumination needed to excite cones. Scotropic vision functions at low levels of illumination, relies on rods and gives a less clear image that is only in black and white. Scotropic vision is usually not funationally during the day due to the bleaching of rhodopsin that occurs with high levels of illumination and the time that it takes to regenerate rhodopsin. However, scotropic vision is highly sensitive at low levels of light due the low detection threshold of rods. Thus, rods enable nocturnal species to have a finely tuned visual motion detection due to the sensitivity and low threshold of rod photoreceptor cells. [28]
In many diurnal species there is a large amount of retinal summation of the rod photoreceptor cells. This is both advantageous and disadvantageous in different ways. The increases summation creates a less clear picture due to the the larger secondary receptive field. Essentially the eye cannot distinguish which specific rods were excited, but only the area in which they synapse with their secondary receptors. However, this large degree of summation also allows for greater sensitivity. The larger primary receptive field allows for a greater amount of summation between all of the rods within the secondary receptive field to sum their individual signals onto the secondary receptive field. However, nocturnal animals deal with this issue by having smaller degree of retinal summation of rod photoreceptors enabling them to have a clearer image and better motion detection. Nocturnal animals also do not have to face the trade-off that diurnal species do as mentioned above because nocturnal animals have more sensitive rods. (see “rods and cones” under general anatomy.) Thus, nocturnal animals can resolve clearer images and detect motion better by having a low degree of retinal summation in addition to having more sensitive rod photoreceptors. [29]
Neuronal Processing
Higher Order Visual Perception
Evolutionary Significance
Evolution to Nocturnality
Nocturnality is a form of crypsis, an adaptation to avoid or enhance predation. In the case of the lion, hunting at night is a large advantage because the lions’ prey cannot see well at night, making it an easy target. In the case of prey, such as rodents and sea turtles, are active at night in order to avoid their diurnal (active during the day) predators. This reduces the risk of predation to both parent and offspring.
It is suggested that nocturnality is also evolutionarily beneficial in competition for resources and predation. For example, hawks and owls are in direct competition for the same resources,but, because the owl is nocturnal and the hawk is diurnal so they can hunt the same field, meadow or forest with the same rodents with virtually no conflict.
One reason for nocturnal adaption of animals in arid biomes and deserts may be for the conservation of water and avoidance of heat. This adaptation enhances osmoregulation (the active regulation of osmotic pressure of an organisms fluids to maintain homeostasis of the organisms water content).[1]
Evolution from Nocturnality
Some paleontological records of mammals are interpereted as at one point in their early history all mammals having had nocturnal vision. The most prominent evidence supporting this theory is that most mammals are limited to dichromacy, meaning most mammals have only two cone receptors. Trichromatic mammals, such as diurnal primates, have only recently developed a third cone opsin gene. These genes probably developed for the advantageous color recognition of food.
However, a recently developed third cone and that most mammals are dichromatic is not the only evidence for most mammals being nocturnal. The retinas of mammals are flooded with rods (especially in comparison with other taxa) which shows favor of increased light sensitivity over visual accuity pointing to mammals evolution in primarily low light environments.
The most important determining factor of the organization of the visual apparatus is activity pattern. Activity pattern determines the amount of light available to an animals visual apparatus and determines optical demands of imaging under more or less light and requires different eye shapes. Comparative studies of eye size and shape between diurnal birds and lizards and nocturnal birds and lizards shows correlation between eye shape and activity pattern. Nocturnal birds and lizards have larger corneal diameters relative to the axial lengths of the eye (possibly to allow more light to enter, increasing visual sensitivity). Conversely, diurnal birds and lizards have larger axial length of the eye compared to a smaller corneal diameter. An increased axial length allows light to travel farther within the eye and increases the amount of photoreceptors over which an image is projected.
This pattern of eye shape and size changes in relation to the availability of light and is also observed in primates, but not necessarily found in non-primate mammals. Instead of a change in eye shape and size across non-primate mammals, all non-primate mammals have a nocturnal eye shape regardless of activity pattern.
After the mammalian eye had adapted to low-light conditions, the characteristic shape of nocturnal eye shape was maintained even through evolution of multiple diurnal lineages. Diurnal owls and geckos maintained a nocturnal eye shape with the larger corneal diameter relative to axial length.[30]
Nocturnal Animals of Interest
Proposed Exam Questions
- Name three reasons why evolution of nocturnal vision is advantageous?
- What is the advantage to Nocturnal animals in having a higher percentage of rods in the retina?
- What is the function of the tapetum lucidum in nocturnal mammals?
References
- ↑ 1.0 1.1 1.2 1.3 Wikipedia - Nocturnal
- ↑ Wikipedia - Night Vision - Biological Night Vision
- ↑ The Nocturnal Eye http://www.pbs.org/wgbh/nova/kalahari/nocturnaleye.html]
- ↑ [Warrant, E. J. (1999). Seeing better at night: life-style, eye design and the optimum strategy of spatial and temporal summation. Vision Res. 39, 1611- 1630.]
- ↑ 5.0 5.1 Tim Malmström and Ronald H. H. Kröger. Pupil shapes and lens optics in the eyes of terrestrial vertebrates. http://jeb.biologists.org/content/209/1/18.full.pdf
- ↑ Wild Cat Facts & FAQ http://ds.dial.pipex.com/agarman/bco/fact4.htm
- ↑ Molly Kirk and David Denning. How do animals see in the dark? http://www.ebiomedia.com/how-do-animals-see-in-the-dark.html
- ↑ Beier, Paul. Effects of Artificial Night Lighting on Terrestrial Mammals. http://oak.ucc.nau.edu/pb1/vitae/Beier-Lights-Mammals-FINAL[w.%20my%20edits].pdf
- ↑ Solovei, I., Kreysing, M., Lanctôt, C., Kösem, S., Peichl, L., Cremer, T., Guck, J., & Joffe, B. (2009). Nuclear Architecture of Rod Photoreceptor Cells Adapts to Vision in Mammalian Evolution Cell, 137 (2), 356-368 DOI: 10.1016/j.cell.2009.01.052
- ↑ Kern, Thomas. Tapetum causes dogs' eyes to reflect in different colors. http://www.ccmr.cornell.edu/education/ask/?quid=597
- ↑ Antionette, Pirie Nature, Volume 183, Issue 4666, pp. 985-986 (1959).
- ↑ Schmitz, L., & Motani, R. Morphological differences between the eyeballs of nocturnal and diurnal amniotes revisited from optical perspectives of visual environments. Vision Research (2010), doi:10.1016/j.visres.2010.03.009
- ↑ Tarsiers - The Big-Eyed, Ancient, Nocturnal Mammal http://www.factzoo.com/mammals/tarsiers.html
- ↑ Third Eyelid. Web. December, 5 2011. http://www.vetmed.ucdavis.edu/courses/vet_eyes/conotes/con_chapter_5.html
- ↑ Roots, Clive. Nocturnal Animals. Print. Greenwood Publishing 2006.
- ↑ 16.0 16.1 http://en.wikipedia.org/wiki/Tapetum_lucidum#cite_note-Ollivier.2B2004-2 Wikipedia – Tapetum Lucidum
- ↑ http://www.brain.mpg.de/research/research-units/comparative-anatomy-of-the-mammalian-retina/research/
- ↑ http://en.wikipedia.org/wiki/Photoreceptor_cell
- ↑ http://en.wikipedia.org/wiki/Visual_phototransduction
- ↑ http://www.ncbi.nlm.nih.gov/books/NBK10806/
- ↑ http://www.ratbehavior.org/RatVision.htm
- ↑ 22.0 22.1 Daryi Wang, Todd Oakley, Jeffrey Mower, Lawrence C. Shimmin, Sokchea Yim, Rodney L. Honeycutt, Hsienshao Tsao,k and Wen-Hsiung Li. Molecular Evolution of Bat Color Vision Genes. http://mbe.oxfordjournals.org/content/21/2/295.full.pdf
- ↑ Almut Kelber* and Lina S. V. Roth. Nocturnal colour vision – not as rare as we might think. http://jeb.biologists.org/content/209/5/781.full.pdf
- ↑ Huabin Zhao, Stephen J. Rossiter, Emma C. Teeling, Chanjuan Li, James A. Cotton, and Shuyi Zhang. The evolution of color vision in nocturnal mammals. http://www.pnas.org/content/106/22/8980.full.pdf?with-ds=yes
- ↑ Gerald H. Jacob. Evolution of colour vision in mammals. http://rstb.royalsocietypublishing.org/content/364/1531/2957.full.pdf
- ↑ 26.0 26.1 26.2 http://www.neitzvision.com/content/publications/1993-Jacobs-Photopigments_and_color_vision_in_aotus-VisRes.pdf
- ↑ http://www.mapoflife.org/topics/topic_571_Monochromacy-in-mammals/
- ↑ The Eye and Night Vision http://www.aoa.org/x5352.xml
- ↑ Kay RF, Kirk EC. Osteological evidence for the evolution of activity pattern and visual acuity in primates. Am. J. Phys. Anthropol. 2000;113:235–262. [PubMed] http://www.ncbi.nlm.nih.gov/pubmed/11002207
- ↑ http://0-search.ebscohost.com.cals.evergreen.edu/login.aspx?direct=true&db=a9h&AN=53025524&site=ehost-live